Irrigation reduction resistance mechanisms in the rapid fruit growth stage of pears (Pyrus communis L.)
Mecanismos de resistencia en la reducción del riego en la fase de rápido crecimiento del fruto de pera (Pyrus communis L.)
DOI:
https://doi.org/10.15446/agron.colomb.v33n3.52573Keywords:
evapotranspiration, irrigation rates, drought resistance, physiological adaptation, cell walls, pome fruits (en)evapotranspiración, dosis de riego, resistencia a la sequía, adaptación fisiológica, pared celular, pomáceas (es)
Downloads
Doi: 10.15446/agron.colomb.v34n1.52573
1 Department of Civil and Agricultural Engineering, Universidad Nacional de
Colombia. Bogota (Colombia). jevelezs@unal.edu.co
2 Department of Plant Physiology-Biochemistry,
Instituto Nacional de Ciencias Agricolas (INCA). San Jose de Las Lajas (Cuba)
Received for publication: 20 August, 2015. Accepted for publication: 28 March, 2016.
ABSTRACT
Plants adopt physiological defense mechanisms to counteract droughts. In Colombia, there is no information for these mechanisms in pears (Pyrus communis L.), cv. Triunfo de Viena. Therefore, the present study aimed to analyze the hydric conditions of this plant in order to determine if it has developed a mechanism to avoid, reduce, or tolerate water stresses as a defense. This experiment used a pear crop located in the municipality of Sesquile (Colombia), with 16-year-old trees that were subjected to three irrigation treatments: a control that received 100% of this crop's evapotranspiration (100%ETc), and 73%ETc and 53%ETc treatments were irrigated at 73% and 53% of crop's evapotranspiration, respectively, for the entire rapid fruit growth period of 2012 to 2013. The results indicated that the irrigation reduction in 53%ETc treatment present an adaptive mechanism in the trees, with a structural change in the cellular wall that allowed for 47% water savings in this fruit growth stage.
Key words: evapotranspiration, irrigation rates, drought resistance, physiological adaptation, cell walls, pome fruits.
RESUMEN
Las plantas adoptan mecanismos fisiológicos de defensa para enfrentar la sequía y en Colombia no existe información sobre estos mecanismos en pera (Pyrus communis L.) cv. Triunfo de Viena. Por esta razón, el propósito del presente estudio fue analizar las condiciones hídricas de la planta con el fin de determinar si desarrolla algún mecanismo de resistencia para evitar, retrasar o tolerar en respuesta a un estrés hídrico. El experimento se realizó en un cultivo de pera ubicado en el municipio de Sesquile (Colombia), en árboles de 16 años de edad, que fueron sometidos a tres tratamientos de riego. Un tratamiento control (100%ETc) regado con el 100% de la evapotranspiración del cultivo durante todo el ciclo, y los tratamientos 73%ETc y 53%ETc que fueron regados a 73% y 53% de la evapotranspiración del cultivo, respectivamente, durante el periodo de crecimiento rápido del fruto en el periodo de 2012 al 2013. Los resultados obtenidos mostraron que la reducción de riego en el tratamiento 53%ETc se presenta un mecanismo de adaptación en los árboles, mediante un cambio estructural de la pared celular, permitiendo ahorros de agua de un 47% en esta etapa de crecimiento del fruto.
Palabras clave: evapotranspiración, dosis de riego, resistencia a la sequía, adaptación fisiológica, pared celular, pomáceas.
Introduction
The hydric condition of plants is an important factor due to the function of water in all of the biochemical and physiological processes of growth and development (Ortiz, 2006). The state of a plant reflects the conditions of the soil and the effects of the climate (Cohen et al., 2001; Vélezet al., 2007a).
A decrease in the edaphic moisture and an increase in the evaporative demand unleash a series of events that starts with a decrease in the water potential (Ψ), which is seen in all of the plant organs, causing cellular turgor loss (Ψp); followed by a synthesis of abscisic acid (ABA), blockage of the synthesis of cytokinins in the roots, an increase in the xylematic sap (Vila, 2011), a decrease in foliar expansion, stomatal closure, peroxidation of lipids, changes in the permeability of the membranes, degradation of the proteins and changes in gene expression (Moreno, 2009).
Long drought periods can induce anatomical modifications in plants. Changes in the mesophyll structure of olive and avocado leaves have been observed with hydric stresses, with the start of the drought resulting in a rapid modification of the tree-water ratio, decreasing the water potential of the leaves or stems (Morandi et al., 2014). These changes can affect the water potential gradient between the different tree organs with consequences in the xylem and phloem. At the foliar level, ABA and other molecules are transported from the roots for use as signals to reduce stomatic conductance and avoid water loss through excessive transpiration (Morandi et al., 2014).
A multitude of drought resistance mechanisms exist in plants. Evolution has resulted in the development of different responses and adaptations that allow for survival in conditions of constant water deficiency (Moreno, 2009). The principal mechanisms are: escape, avoidance, delay or tolerance to water stress, resulting in adaptation and survival (Cruz et al., 2012).
In the escape mechanism, the plant reduces the vegetative cycle and restricts it to the non-drought period, entering dormancy and adapting to very brief periods with edaphic moisture (Torrecillas et al., 1996).
The avoidance mechanisms act on distinct points of the water transport system in the plant and physical action, facilitating the acquisition of water or limiting its loss. To avoid hydric stress, the avoidance mechanisms minimize resistance to the flow of water in the plant, limit transpiration, or promote a reduction in the osmotic potential in order to favor the uptake of symplastic water; furthermore, physical changes occur, such as stomatal closure, increases in water conductance, changes in the tissue elasticity, and morphological changes that decrease the absorbed radiation in order to minimize transpiration (leaf folding, development of impermeable cuticles and hair) (Vila, 2011).
The resistance mechanisms are biochemical in nature and act at the cellular level; tissues suffer the stress and develop biochemical and morphological transformations to counteract it. The morphological transformations result in a decrease in the foliar area, leaf folding or anthocyanin coating, and doubling of the cellular wall and vacuoles that support the cell. Meanwhile, the chemical transformations release thermal energy (xanthophyll cycle) and facilitate the synthesis of compatible solutes, synthesis of antioxidants, and synthesis of antioxidant enzymes (Vila, 2011).
A plant's hydric stress can be monitored through the water potential (Ψ), expressed in megapascals (MPa). It reflects the water tension in the conduction vacuoles of the plant, defining the stress level of the plant (Muñoz, 2005; Arévalo-H. et al., 2013). Another way to look at the hydric state and the mechanisms of adaptation in plants is the volume pressure curve; this technique provides complete information of the hydric state of the leaf, allowing for a determination of a broad spectrum of water parameters in the tissues, such as the total water content, turgor weight/dry weight ratio, relative water content (RWC), apoplastic water content, symplastic water content, osmotic pressure at zero turgidity and the mean elasticity model (Polaníaet al., 2003).
The present study aimed to analyze the hydric conditions of a pear crop (Pyrus communis L., cv. Triunfo de Viena) in order to determine if it has developed a mechanism to avoid, reduce, or tolerate water stresses as a defense.
Materials and methods
This experiment was carried out between October of 2012 and March of 2013 with a pear crop (P. communis), Triunfo de Viena variety, planted in 1998 with a 4 x 4 m pattern and drip irrigation with six emitters per plant (each giving 8 L h-1) and a frequency of 2 d in a 0.32 ha lot with 17 rows of 10 trees each on the San Benito Farm in the Boitiva district of the municipality of Sesquile (Colombia), at 5º02'53.65" N and 73º48'12.78" W and an altitude of 2,595 m a.s.l., with a mean annual temperature of 14ºC (Molina-Ochoa et al., 2015).
Three irrigation treatments were evaluated: a control treatment (100%ETc) with 100% of the crop evapotranspiration (ETc) throughout the year and 73%ETc and 53%ETc treatments with 73% and 53% of the ETc, respectively, throughout the rapid fruit growth period of 2012 to 2013.
A completely random block design was used with three treatments and four replications per treatment (12 lots). Two trees were randomly selected for each lot, taking into account the border effect.
The hydric balance was determined according to the water requirements of the crop, taking into account the mean monthly precipitation, effective precipitation, potential evapotranspiration of the crop and the crop coefficient (Kc) according to the Allen et al. (2006), in accordance with the climatic information that was obtained with a portable WS-GP1 weather station (ATdelta-T Devices, Cambridge, UK), installed beside the experiment lot. The climate of the region is temperate with moderate rain throughout the year, with a mean temperature of 14ºC and annual precipitation of 890 to 1,500 mm, concentrated in the months of April to May and October to November.
Quantifying the water content and evolution of the soil as a result of rain and irrigation allows for irrigation programming and reveals the effects of water suppression or deficits in the stages of the physiological cycle of the crop (Vélezet al., 2007a). In order to discover the water potential matrix of the soil (Ψs), 10 granular matrix sensors per treatment were used for the measurements (Watermark Mod. 200ss Irrometer Co., Riverside, CA), installed at a depth of 30, 26 cm from the emitter and drip lines, to avoid directly wetting the drip irrigation emitter.
The hydric state of the plants was determined through the stem water potential (Ψt), using a Scholander pressure chamber (Model 600, PMS Instrument Co., Corvallis, OR) at midday every 15 d, following the procedure described by Scholander et al. (1965), in three mature leaves on two trees per repetition, for a total of 24 per treatment, which were located on the northern side of the trees and covered with aluminum-wrapped, hermetic plastic bags for 2 h before the measurement in order to prevent transpiration.
In order to observe the daily evolution of the foliar water potential of the pear crop (P. communis), 75 days after full (DAF), four leaves with a normal transpiration state were collected per repetition, for a total of 16 leaves in the 100% ETc treatment, starting the measurement before sunrise (4:00 AM) and repeating it every 2 h until the potential recovery was observed in the plant (7:00 PM).
In order to reveal the drought resistance mechanisms that the pear trees have adapted, the volume pressure curve was graphed with measurements of the water restriction. For this, 20 completely developed leaves were selected and cut per repetition and immediately hydrated for 24 h in a dark chamber in order to saturate them and observe the total turgidity at the time of measurement. After 24 h, the water potential was determined with a Scholander pressure chamber and the fresh weight was determined with an electronic precision balance, X73%ETC20A (0.001 g precision). This procedure was repeated successively during the dehydration of the tissue in the natural environmental conditions until the variations in the water loss and water potential were minimal (Cruz et al., 2012).
At the end of this process, the leaves were dried in an oven at 80ºC for 48 h in order to determine the dry weight and, finally, the relative water content (RWC, %) (Eq. 1) and the total water content (WC, %) (Eq. 2) were calculated.
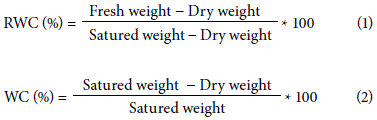
In order to obtain the saturated foliar osmotic potential (Ψos), fourth leaves were selected per repetition, wrapped in aluminum foil, and frozen at -50ºC with liquid nitrogen to detect the metabolic activity (Azcón-Bieto and Talón, 2008). In order to measure the Ψos, the leaves were thawed and centrifuged with an International device for 10 min at 10,000 rpm to obtain the cellular juice to determine the Ψos with a Wescor 5520 vapor pressure osmometer (Wescor Inc., Logan, UT).
The inverse of the leaf water potential (Ψ) was graphed against the relative water content (RWC), obtaining the volume pressure curve for each replication of the three treatments for the evaluated period (Fig. 1).

The modified hyperbolic equation II was used to fit the curve (Eq. 3).

Where, f is the inverse of the potential in MPa, x is the relative water content, a and b are the regression parameters.
A dotted, straight line was graphed, separating the point of the observed 1/Ψos, intercepting the curve and extending to the %RWC axis point where 1/Ψos was zero (Rodríguez et al., 2012) because the dependence of Ψos on the cellular volume was approximately linear (Azcón-Bieto and Talon, 2008), in order to determine the inverse of the osmotic potential at the turgor loss point (1/Ψoptlp), the relative apoplastic water content (RWCa), and the elasticity model (Є), which indicated the rigidity of the cellular walls, which, when higher, results in higher resistance to deformation in the wall (Azcón-Bieto and Talón, 2008), as determined with Eq. 4 from Patakas and Nortsakis (1999).

To determine the influence of the irrigation restriction on the fruit setting, a quantitative evaluation was carried out (Garzónet al., 2013). The BBCH scale was used for the selection criteria (Bleiholder, 1996). Principal stage 5 (appearance of floral organs) was selected, code 55 (female flowers, visible (still closed)). In November, two branches were selected and marked at the cardinal point (eight per tree) in two trees per replication, for a total of 64 per treatment, counting the number of flowers every 15 d until the fruit harvest.
The results were analyzed with Statistics IBM (IBM Corp. Released, 2011) through an analysis of variance (ANOVA) and a Duncan mean comparison test, with a 5% significance level.
Results
During the restriction period, the mean daily maximum and minimum temperatures were 15.37ºC (79 DAF) and 10.71ºC (77 DAF), respectively. The daily vapor pressure deficit (VPD) values had a range of 0.183 and 0.696 KPa at 58 and 94 DAF, respectively, demonstrating a tendency to increase in the days with higher temperatures, as expected. The daily ETo demonstrated an oscillating behavior with a tendency to increase starting at 20 DAF, decreasing with precipitation, as seen between 51 and 58 DAF, as well as at 107 DAF, when there was a new tendency to decrease for the same reason (Fig. 2).
During the irrigation restriction in the experiment period, the water potential matrix in the soil (Ψs) (Fig. 3) reached minimum values of -111.40; -123.00 KPa at 119 DAF and -145.40 KPa at 106 DAF in treatments 100%ETC, 73%ETC and 53%ETC, respectively. The lower Ψs values were seen in the treatment with the biggest reduction of the water lamina (53%ETC), with significant differences (P≤0.05) when compared to the properly irrigated treatment (100%ETC) throughout the period, except at 53, 147 and 161 DAF, due to precipitation that prompted recovery and leveling of the treatments. These results corroborated the sensitivity and precision of the sensors that were used to monitor the soil moisture in this experiment for the drying cycle. In a general sense, the Ψs can be defined as having had an expected behavioral relationship with the irrigation application, with recovery when the rains arrived, reaching a balance at 161 DAF, corresponding to the difference of the potential matrix of the soil in accordance with the applied irrigation lamina.

During the irrigation restriction, the Ψt (Fig. 4) demonstrated a behavior that was similar in all of the treatments, with some exceptions in the treatment with the lowest water lamina (53%ETC) when there was no precipitation. The higher values were seen at the start of the irrigation restriction.

Treatment 53%ETC, which had the lowest irrigation lamina, had the lowest Ψt value (-1.05 MPa) and the control (100%ETC) had the highest (-0.96 MPa) at 106DAF; these values are lower than the ones observed by Molina (2014) in the same lot during the 2011 to 2012 cycle, applying irrigation lamina that corresponded to 67 and 55% of the ETc, with a mean Ψt of -0.65 and -0.42 MPa, respectively.
During the 87 d of irrigation restriction, the Ψt decreased similarly in the three treatments, with recovery when the precipitation was 17.80; 6.20; 1.00 and 1.00 mm at 107, 109, 110 and 111 DAF, respectively, with values between -0.93 and -0.95 MPa (119 DAF). The stem potential values (Ψt) during the experiment were considered typical for fruit trees with these characteristics when properly irrigated. Similar results were obtained by Galindo et al. (2014) and Rodríguez et al. (2012) in pomegranate, by Cruz et al. (2012) in jujube (Zizyphus jujuba), and by Mellisho et al. (2011) when studying the hydric stress resistance mecha- nisms in peach trees exposed to different irrigation levels in the Mediterranean region. These studies were carried out in the rapid fruit growth phase. Morandi et al. (2014) observed a Ψt range of -1.22 to -0.30 MPa in pears, Abbèfétel variety, with a 25% ETc application, results that agree with those of the present study, independent of the applied treatments.
Figure 5 shows the daily evolution of the foliar water potential (Ψ) in the control treatment (100%ETC) under suitable irrigation conditions, which were directly proportional to the evaporative demand, oscillating between -0.22 and -1.42 MPa between 4:00 and 14:00, gradually decreasing in the morning, stabilizing at midday and starting recovery at 14:00; the same behavior was observed in the Mediterranean climate by Cruz et al. (2012) in Z. jujuba and by
Vélez et al. (2007b) in Nules clementine.

It was also observed that the foliar water potential (Ψ) had an inverse relationship with the vapor pressure deficit, with higher Ψ values when the VPD decreased (Fig. 4), similar results were found by Measham et al. (2014) in sweet cherries (Prunus avium); by Vélezet al. (2007a) in clementines; by Morandi et al. (2014) in the pear variety AbbéFétel and by Molina (2014) in the same crop.
Figure 6 presents the volume pressure curve for the three treatments, plotted with the mean values that were obtained in each replication with the modified hyperbolic equation II and the coefficient of significance determination (R2) for each of the treatments.

The osmotic potential (Ψos) (Tab. 1) was not affected by the irrigation lamina, with values of -2.29; -2.36 and -2.36 MPa for 100%ETC, 73%ETC and 53%ETC, respectively, without significant differences (P≤0.05) between the treatments.
The isothermal volume pressure of the replications of each treatment was used to obtain the leaf water content (LWC), the relative water content at the turgor loss point (RWCtlp), the relative apoplastic water content (RWCa), the osmotic potential at the turgor loss point (Ψoptlp) and the maximum elasticity (Єmax) (Tab. 1).

The osmotic potential at the turgor loss point (Ψoptlp) did not have significant differences (P≤0.05) between the treatments, which indicated that the plants did not exhibit a tolerance mechanism that involved adjusting the osmotic potential in order to maintain the water gradient from the soil to the leaves, possibly because the hydric stress did not occur for a prolonged period and was not very severe (Cruz et al., 2012; Arndt et al., 2001); in this case, the restriction period to the measurement point was only 92 DAF.
The Єmax values were similar between the treatments, without significant differences (P≤0.05), which indicated that an elasticity adjustment response to the hydric stress was not developed, a similar behavior to that seen for the RWCtlp. Savéet al. (1995) confirmed that the RWCtlp is directly influenced by the Єmax.
It is important to note the significant difference (P≤0.05) observed between the control treatment (100%ETC) and 53%ETC for the relative apoplastic water content (RWCa), which can be interpreted as an adaption mechanism for hydric stresses (Serrano and Peñuelas, 2005) because there was a structural change in the cellular wall that allowed for a higher accumulation of apoplastic water (Cruz et al., 2012). The RWCa values were high (49-60%) when com- pared to those observed by Cruz et al. (2012) in jujube (Z. jujuba) (29-41%) and by Rodríguez et al. (2012) in pomegranate (41-54%); this may have been due to the semiarid climate of the crops or to the high resistance to hydric deficits in this species.
There were no significant differences (P≤0.05) between the treatments for the percentage of fruit set or physiological fruit drop, which indicates that the restriction applied during the 2011 to 2012 cycle with irrigation lamina that corresponded to 67 and 55% of the ETc for treatments 73%ETC and 53%ETC did not affect the fruit set or drop in the second year of the applied restrictions (Fig.7). Similar results were reported by Pierantozzi et al. (2013) in olive trees (Olea europea L. cvs. Arbequina and Manzanilla) with irrigation lamina applications that were 75 and 50% of the ETc for 2 years.

The percentage of fruit drop was 12.72, 16.79 and 13.77% for treatments 100%ETC, 73%ETC, and 53%ETC, respectively. The physiological fruit drop was higher in the more deficient treatment (40%) as compared to the control treatment (21%). Girona et al. (2003) found that a hydric stress in peach trees decreases the fruit drop before the last growth phase, similar to the findings of Intrigliolo et al. (2013) for pomegranate fruits that received a regulated deficit irrigation application during the flowering and fruit set periods.
Conclusions
The parameters obtained with the isothermal volume pressure demonstrated a clear methodology for the study of hydric stress tolerance mechanisms in these crops. The pear (P. communis) did not carry out an osmotic adjustment nor did it adjust the elasticity in the treatments that had a reduced irrigation lamina. The leaf water content remained at a similar level, while the relative apoplastic water content (RWCa) presented an increase in the treatment with the lowest irrigation lamina (53%ETC), as compared to the properly irrigated treatment (100%ETC), which can be defined as an adaptation mechanism for P. communis for hydric stresses and explains the hydric state of the trees exposed to the irrigation conditions of 53%ETC.
The differences in the fruit drop and set between the treatment with the least irrigation (53%ETC) and the control treatment (100%ETC) were not significant.
Further studies on the mechanisms adopted by P. communis in other production periods with inferior irrigation lamina are recommended in order to determine if other adjustments are carried out for resistance to hydric deficits.
Acknowledgements
This study was financed by Acqua Irrigación Ltda., the Universidad Nacional de Colombia, project Hermes 20719, and the Capacitación del Instituto Nacional de Ciencias Agrícolas de Cuba (INCA).
Literature cited
Allen, R.G., L.S. Pereira, D Raes, and M. Smith. 2006. Evapotranspiración del cultivo: guías para la determinación de los requerimientos de agua de los cultivos. Estudio FAO Riego y Drenaje No. 56. FAO, Roma.
Arévalo-H., J.J., J.E. Vélez, and J.H. Camacho-Tamayo. 2013. Uso eficiente del agua para el cultivo de rosa cv. Freedom bajo invernadero. Rev. Bras. Eng. Agríc. Ambient. 17, 811-817. Doi: 10.1590/S1415-43662013000800002
Arndt, S.K., S.C. Clifford, W. Wanek, H.G. Jones, and M. Popp. 2001. Physiological and morphological adaptations of the fruit tree Ziziphus rotundifolia in response to progressive drought stress. Tree Physiol. 21, 705-715. Doi: 10.1093/treephys/21.11.705
Azcón-Bieto, J. and M. Talón. 2008. Fundamentos de fisiología vegetal. 2nd ed. McGraw-Hill Interamericana de España, Barcelona, Spain.
Bleiholder, H. (ed.). 1996. Compendio para la identificación de los estadios fenológicos de especies mono- y dicotiledóneas cultivadas: escala BBCH extendida. Centro Federal de Investigaciones Biológicas para Agricultura y Silvicultura (BBA). Limburgerhof, Germany.
Cohen, M., D.A. Goldhamer, E. Fereres, J. Girona, and M. Mata. 2001. Assessment of peach tree responses to irrigation water ficits by continuous monitoring of trunk diameter changes. J. Hort. Sci. Biotechnol. 76, 55-60. Doi: 10.1080/14620316.2001.11511327
Cruz, Z.N., P. Rodríguez, A. Galindo, E. Torrecillas, S. Ondoño, C.D. Mellisho, and A. Torrecillas. 2012. Leaf mechanisms for drought resistance in Zizyphus jujuba trees. Plant Sci. 197, 77-83. Doi: 10.1016/j.plantsci.2012.09.006
Galindo, A., P. Rodríguez, J. Collado-González, Z.N. Cruz, E. Torrecillas, S. Ondoño, M. Corell, A. Moriana, and A. Torrecillas. 2014. Rainfall intensifies fruit peel cracking in water stressed pomegranate trees. Agric. For. Meteorol. 194, 29-35. Doi: 10.1016/j.agrformet.2014.03.015
Garzón C., D.L., J.E. Vélez S., and J.O. Orduz R. 2013. Efecto del déficit hídrico en el crecimiento y desarrollo de frutos de naranja Valencia (Citrus sinensis Osbeck) en el piedemonte del Meta, Colombia. Acta Agron. 62, 136-147.
Girona, J., M. Mata, A. Arbonès, S. Alegre, J. Rufat, and J. Marsal. 2003. Peach tree response to single and combined regulated deficit irrigation regimes under shallow soils. J. Amer. Soc. Hort. Sci. 128, 432-440.
IBM Corp. Released. 2011. IBM SPSS statistics for Windows, version 20.0. Armonk, NY.
Intrigliolo, D.S., L. Bonet, P.A. Nortes, H. Puerto, E. Nicolas, and J. Bartual. 2013. Pomegranate trees performance under sustained and regulated deficit irrigation. Irrigation Sci. 31, 959-970. Doi: 10.1007/s00271-012-0372-y
Measham, P.F., S.J. Wilson, A.J. Gracie, and S.A. Bound. 2014. Tree water relations: flow and fruit. Agr. Water Manage. 137, 59-67. Doi: 10.1016/j.agwat.2014.02.005
Mellisho, C.D., Z.N. Cruz, W. Conejero, M.F. Ortuño, and P. Rodríguez. 2011. Mechanisms for drought resistance in early maturing cvar Flordastar peach trees. J. Agric. Sci. 149, 609-616. Doi: 10.1017/S0021859611000141
Molina O., M.J. 2014. Efecto de la aplicación de tres láminas de riego en un cultivo de pera variedad Triunfo de Viena (Pyrus communis L.). MSc thesis. Faculty of Engineering, Universidad Nacional de Colombia, Bogota.
Molina-Ochoa, M.J., J.E. Vélez-Sánchez, and P. Rodríguez. 2015. Efecto del riego deficitario controlado en las tasas de crecimiento del fruto de pera (Pyrus communis L.), var. Triunfo de Viena. Rev. Colomb. Cienc. Hortic. 9, 234-246. Doi: 10.17584/rcch.2015v9i2.4179
Morandi, B., P. Losciale, L. Manfrini, M. Zibordi, S. Anconelli, F. Galli, E. Pierpaoli, and L.C. Grappadelli. 2014. Increasing water stress negatively affects pear fruit growth by reducing firts its xylem and then its phloem inflow. J. Plant Physiol. 171, 1500-1509. Doi: 10.1016/j.jplph.2014.07.005
Moreno F., L.P. 2009. Respuesta de las plantas al estrés por déficit hídrico. Una revisión. Agron. Colomb. 27, 179-191.
Muñoz M., M.V. 2005. Respuesta de la variación del diámetro de tronco y del potencial hídrico xilemático a diferentes regímenes de riego en uva de mesa, cv. Crimson seedless. Undergraduate thesis. Faculty of Agricultural Sciences, Universidad de Chile, Santiago.
Ortiz O., M. 2006. Respuestas fisiológicas y bioquímicas de dos especies de pinos en condiciones limitantes de humedad. Undergraduate thesis. Institute of Agricultural Sciences, Universidad Autónoma del Estado de Hidalgo, Tulancingo de Bravo, Mexico.
Patakas, A. and B. Nortsakis. 1999. Mechanisms involved in diurnal changes of osmotic potential in grapevines under drought conditions. J. Plant Physiol. 154, 767-774. Doi: 10.1016/S0176-1617(99)80256-9
Pierantozzi, P., M. Torres, R. Bodoira, and D. Maestri. 2013. Water relations, biochemical - physiological and yield responses of olive trees (Olea europaea L. cvs. Arbequina and Manzanilla) under drought stress during the pre-flowering and flowering period. Agr. Water Manage. 125, 13-25. Doi: 10.1016/j.agwat.2013.04.003
Polanía, J.A., S. Mejía de T., and L. Rodríguez A. 2003. Curva presión volumen de la caña de azúcar variedad CC 8592 en condiciones del Valle del Cauca. Acta Agron. 52, 71-76.
Rodríguez, P., C.D. Mellisho, W. Conejero, Z.N. Cruz, M.F. Ortuño, A. Galindo, and A. Torrecillas. 2012. Plant water relations of leaves of pomegranate trees under different irrigation conditions. Environ. Exp. Bot. 77, 19-24. Doi: 10.1016/j.envexpbot.2011.08.018
Savé, R., C. Biel, R. Domingo, M.C. Ruiz-Sánchez, and A. Torrecillas. 1995. Some physiological and morphological characteristics of citrus plants for droughet resistance. Plant Sci. 110, 167-172. Doi: 10.1016/0168-9452(95)04202-6
Scholander, P.F., E.D. Bradstreet, E.A. Hemmingsen, and H.T. Hammel. 1965. Sap pressure in vascular plants. Science 148, 339-346. Doi: 10.1126/science.148.3668.339
Serrano, L. and J. Peñuelas. 2005. Contribution of physiological and morphological adjustments to drought resistance in two Mediterranean tree species. Biol. Plant. 49, 551-559. Doi: 10.1007/s10535-005-0049-y
Torrecillas, A., J.J. Alarcón, R. Domingo, J. Planes, and M.J. Sánchez-Blanco. 1996. Strategies for drought resistance in leaves of two almond cultivars. Plant Sci. 118, 135-143. Doi: 10.1016/0168-9452(96)04434-2
Vélez, J.E., D.S. Intringliolo, and J.R. Castel S. 2007a. Programación de riego en citricos con base en sensores de medida del estado hídrico del suelo y de la planta. Ing. Agua 14, 127-136. Doi: 10.4995/ia.2007.2907
Vélez, J.E., D.S. Intrigliolo M., and J.R. Castel S. 2007b. Programación de riego deficitario en clementina de Nules, mediante dendrómetros. Levante Agríc. 387, 313-317.
Vila, H.F. 2011. Regulación de la hidratación y la turgencia foliares por mecanismos evitadores del estrés, y resistencia a déficit hídrico en vid modelo vs. experimento. PhD thesis. Graduate Program in Biology, Universidad Nacional de Cuyo, Mendoza, Argentina.
References
Allen, R.G., L.S. Pereira, D Raes, and M. Smith. 2006. Evapotranspiración del cultivo: guías para la determinación de los requerimientos de agua de los cultivos. Estudio FAO Riego y Drenaje No. 56. FAO, Roma.
Arévalo-H., J.J., J.E. Vélez, and J.H. Camacho-Tamayo. 2013. Uso eficiente del agua para el cultivo de rosa cv. Freedom bajo invernadero. Rev. Bras. Eng. Agríc. Ambient. 17, 811-817. Doi: 10.1590/S1415-43662013000800002
Arndt, S.K., S.C. Clifford, W. Wanek, H.G. Jones, and M. Popp. 2001. Physiological and morphological adaptations of the fruit tree Ziziphus rotundifolia in response to progressive drought stress. Tree Physiol. 21, 705-715. Doi: 10.1093/treephys/21.11.705
Azcón-Bieto, J. and M. Talón. 2008. Fundamentos de fisiología vegetal. 2nd ed. McGraw-Hill Interamericana de España, Barcelona, Spain.
Bleiholder, H. (ed.). 1996. Compendio para la identificación de los estadios fenológicos de especies mono- y dicotiledóneas cultivadas: escala BBCH extendida. Centro Federal de Investigaciones Biológicas para Agricultura y Silvicultura (BBA). Limburgerhof, Germany.
Cohen, M., D.A. Goldhamer, E. Fereres, J. Girona, and M. Mata. 2001. Assessment of peach tree responses to irrigation water ficits by continuous monitoring of trunk diameter changes. J. Hort. Sci. Biotechnol. 76, 55-60. Doi: 10.1080/14620316.2001.11511327
Cruz, Z.N., P. Rodríguez, A. Galindo, E. Torrecillas, S. Ondoño, C.D. Mellisho, and A. Torrecillas. 2012. Leaf mechanisms for drought resistance in Zizyphus jujuba trees. Plant Sci. 197, 77-83. Doi: 10.1016/j.plantsci.2012.09.006
Galindo, A., P. Rodríguez, J. Collado-González, Z.N. Cruz, E. Torrecillas, S. Ondoño, M. Corell, A. Moriana, and A. Torrecillas. 2014. Rainfall intensifies fruit peel cracking in water stressed pomegranate trees. Agric. For. Meteorol. 194, 29-35. Doi: 10.1016/j.agrformet.2014.03.015
Garzón C., D.L., J.E. Vélez S., and J.O. Orduz R. 2013. Efecto del déficit hídrico en el crecimiento y desarrollo de frutos de naranja Valencia (Citrus sinensis Osbeck) en el piedemonte del Meta, Colombia. Acta Agron. 62, 136-147.
Girona, J., M. Mata, A. Arbonès, S. Alegre, J. Rufat, and J. Marsal. 2003. Peach tree response to single and combined regulated deficit irrigation regimes under shallow soils. J. Amer. Soc. Hort. Sci. 128, 432-440.
IBM Corp. Released. 2011. IBM SPSS statistics for Windows, version 20.0. Armonk, NY.
Intrigliolo, D.S., L. Bonet, P.A. Nortes, H. Puerto, E. Nicolas, and J. Bartual. 2013. Pomegranate trees performance under sustained and regulated deficit irrigation. Irrigation Sci. 31, 959-970. Doi: 10.1007/s00271-012-0372-y
Measham, P.F., S.J. Wilson, A.J. Gracie, and S.A. Bound. 2014. Tree water relations: flow and fruit. Agr. Water Manage. 137, 59-67. Doi: 10.1016/j.agwat.2014.02.005
Mellisho, C.D., Z.N. Cruz, W. Conejero, M.F. Ortuño, and P. Rodríguez. 2011. Mechanisms for drought resistance in early maturing cvar Flordastar peach trees. J. Agric. Sci. 149, 609-616. Doi: 10.1017/S0021859611000141
Molina O., M.J. 2014. Efecto de la aplicación de tres láminas de riego en un cultivo de pera variedad Triunfo de Viena (Pyrus communis L.). MSc thesis. Faculty of Engineering, Universidad Nacional de Colombia, Bogota.
Molina-Ochoa, M.J., J.E. Vélez-Sánchez, and P. Rodríguez. 2015. Efecto del riego deficitario controlado en las tasas de crecimiento del fruto de pera (Pyrus communis L.), var. Triunfo de Viena. Rev. Colomb. Cienc. Hortic. 9, 234-246. Doi: 10.17584/rcch.2015v9i2.4179
Morandi, B., P. Losciale, L. Manfrini, M. Zibordi, S. Anconelli, F. Galli, E. Pierpaoli, and L.C. Grappadelli. 2014. Increasing water stress negatively affects pear fruit growth by reducing firts its xylem and then its phloem inflow. J. Plant Physiol. 171, 1500-1509. Doi: 10.1016/j.jplph.2014.07.005
Moreno F., L.P. 2009. Respuesta de las plantas al estrés por déficit hídrico. Una revisión. Agron. Colomb. 27, 179-191.
Muñoz M., M.V. 2005. Respuesta de la variación del diámetro de tronco y del potencial hídrico xilemático a diferentes regímenes de riego en uva de mesa, cv. Crimson seedless. Undergraduate thesis. Faculty of Agricultural Sciences, Universidad de Chile, Santiago.
Ortiz O., M. 2006. Respuestas fisiológicas y bioquímicas de dos especies de pinos en condiciones limitantes de humedad. Undergraduate thesis. Institute of Agricultural Sciences, Universidad Autónoma del Estado de Hidalgo, Tulancingo de Bravo, Mexico.
Patakas, A. and B. Nortsakis. 1999. Mechanisms involved in diurnal changes of osmotic potential in grapevines under drought conditions. J. Plant Physiol. 154, 767-774. Doi: 10.1016/S0176-1617(99)80256-9
Pierantozzi, P., M. Torres, R. Bodoira, and D. Maestri. 2013. Water relations, biochemical - physiological and yield responses of olive trees (Olea europaea L. cvs. Arbequina and Manzanilla) under drought stress during the pre-flowering and flowering period. Agr. Water Manage. 125, 13-25. Doi: 10.1016/j.agwat.2013.04.003
Polanía, J.A., S. Mejía de T., and L. Rodríguez A. 2003. Curva presión volumen de la caña de azúcar variedad CC 8592 en condiciones del Valle del Cauca. Acta Agron. 52, 71-76.
Rodríguez, P., C.D. Mellisho, W. Conejero, Z.N. Cruz, M.F. Ortuño, A. Galindo, and A. Torrecillas. 2012. Plant water relations of leaves of pomegranate trees under different irrigation conditions. Environ. Exp. Bot. 77, 19-24. Doi: 10.1016/j.envexpbot.2011.08.018
Savé, R., C. Biel, R. Domingo, M.C. Ruiz-Sánchez, and A. Torrecillas. 1995. Some physiological and morphological characteristics of citrus plants for droughet resistance. Plant Sci. 110, 167-172. Doi: 10.1016/0168-9452(95)04202-6
Scholander, P.F., E.D. Bradstreet, E.A. Hemmingsen, and H.T. Hammel. 1965. Sap pressure in vascular plants. Science 148, 339-346. Doi: 10.1126/science.148.3668.339
Serrano, L. and J. Peñuelas. 2005. Contribution of physiological and morphological adjustments to drought resistance in two Mediterranean tree species. Biol. Plant. 49, 551-559. Doi: 10.1007/s10535-005-0049-y
Torrecillas, A., J.J. Alarcón, R. Domingo, J. Planes, and M.J. Sánchez-Blanco. 1996. Strategies for drought resistance in leaves of two almond cultivars. Plant Sci. 118, 135-143. Doi: 10.1016/0168-9452(96)04434-2
Vélez, J.E., D.S. Intringliolo, and J.R. Castel S. 2007a. Programación de riego en citricos con base en sensores de medida del estado hídrico del suelo y de la planta. Ing. Agua 14, 127-136. Doi: 10.4995/ia.2007.2907
Vélez, J.E., D.S. Intrigliolo M., and J.R. Castel S. 2007b. Programación de riego deficitario en clementina de Nules, mediante dendrómetros. Levante Agríc. 387, 313-317.
Vila, H.F. 2011. Regulación de la hidratación y la turgencia foliares por mecanismos evitadores del estrés, y resistencia a déficit hídrico en vid modelo vs. experimento. PhD thesis. Graduate Program in Biology, Universidad Nacional de Cuyo, Mendoza, Argentina.
How to Cite
APA
ACM
ACS
ABNT
Chicago
Harvard
IEEE
MLA
Turabian
Vancouver
Download Citation
License
Copyright (c) 2016 Agronomía Colombiana

This work is licensed under a Creative Commons Attribution-NonCommercial-ShareAlike 4.0 International License.
© Centro Editorial de la Facultad de Ciencias Agrarias, Universidad Nacional de Colombia
Reproduction and quotation of material appearing in the journal is authorized provided the following are explicitly indicated: journal name, author(s) name, year, volume, issue and pages of the source. The ideas and observations recorded by the authors are their own and do not necessarily represent the views and policies of the Universidad Nacional de Colombia. Mention of products or commercial firms in the journal does not constitute a recommendation or endorsement on the part of the Universidad Nacional de Colombia; furthermore, the use of such products should comply with the product label recommendations.
The Creative Commons license used by Agronomia Colombiana journal is: Attribution - NonCommercial - ShareAlike (by-nc-sa)

Agronomia Colombiana by Centro Editorial of Facultad de Ciencias Agrarias, Universidad Nacional de Colombia is licensed under a Creative Commons Reconocimiento-NoComercial-CompartirIgual 4.0 Internacional License.
Creado a partir de la obra en http://revistas.unal.edu.co/index.php/agrocol/.