Identification of resilience factors, variables and indicators for sustainable management of urban drainage systems
Identificación de factores, variables e indicadores de resiliencia para la gestión sostenible de sistemas de drenaje urbano
DOI:
https://doi.org/10.15446/dyna.v84n203.58116Palabras clave:
urban drainage, management, resilience, sustainability (en)drenaje urbano, gestión, resiliencia, sostenibilidad (es)
Descargas
Recibido: 14 de junio de 2016; Revisión recibida: 19 de julio de 2017; Aceptado: 30 de septiembre de 2017
Abstract
Water management systems in general and urban drainage systems (UDS) in particular should be designed to ensure not only the provision of public service but also their sustainability and resilience. This paper performed an analysis and assessment to identify the factors, variables and indicators of resilience for sustainable UDS management by using an information management tool for scientific subjects. As a result of this analysis, four water resource resilience factors were identified: i. Flexibility; ii. Resourcefulness; iii. Redundancy; and iv. Robustness. In addition, six UDS resilience variables were identified: i. Recovery capacity; ii. Response capacity; iii. Amplitude; iv. Absorption capacity; v. Resistance capacity; and vi. Response curve. Corresponding indicators were proposed to quantify these variables. The identified elements contribute to the development of integrated frameworks to assess UDS resilience.
Keywords:
urban drainage, management, resilience, sustainability..Resumen
La gestión del agua en general y de los sistemas de drenaje urbano (SDU) en particular, debe ser concebida no solo para asegurar la prestación de un servicio público, sino también para garantizar su sostenibilidad y resiliencia. En el presente artículo se presenta un análisis y reflexión que permitió identificar los factores, variables e indicadores de resiliencia para la gestión sostenible de SDU, usando herramientas de gestión de información de temas científicos. Como resultado de este análisis, se identificaron cuatro factores de resiliencia de recursos hídricos: i. Flexibilidad; ii. Recursividad; iii. Redundancia; y iv. Robustez y seis variables de resiliencia de SDU: i. Capacidad de recuperación; ii. Capacidad de respuesta; iii. Amplitud; iv. Capacidad de absorción; v. Capacidad de resistencia; y vi. Curva de respuesta. Para cuantificar estas variables, se proponen sus correspondientes indicadores. Los elementos identificados contribuyen al desarrollo de modelos integrales de evaluación de la resiliencia en SDU.
Palabras clave:
drenaje urbano, gestión, resiliencia, sostenibilidad..1. Introduction
Cities must be able to face 21st century threats such as rapid urbanization, population growth, climate change and variability, energy restrictions and increased environmental regulation [1]. Novotny [2] noted that urban water management becomes unsustainable in the face of extreme events such as floods or droughts, which are expected to increase in frequency as a result of global warming.
Accordingly, there is a need to consider resilience in the planning, design and construction of urban infrastructure as embodied in the Sustainable Development Goals (SDGs) formulated by the UN for 2016-2030 [3].
Urban infrastructure elements such as urban drainage systems (UDS) are vulnerable to these aforementioned threats; therefore, design and operational processes must account for system weaknesses, operational failures, and the incorrect interpretation and use of information to ensure sustainability [4].
Thus, the concept of resilience comes to the fore when seeking to improve the sustainability of urban infrastructure in the future.
Given the importance of this concept in UDS management, we will discuss the evolution of resilience, going from the narrowest sense of the word to a broader interpretation, while examining how it has been addressed as it relates to UDS management.
1.1. Evolution of the concept of resilience
The concept of resilience arose from the field of ecology in the 1960s through studies of predator-prey population interactions and their functional responses as they relate to the theory of ecological stability.
Holling [5] proposed that “resilience determines the persistence of relationships within a system and is a measure of the capacity of these systems to absorb changes in variables of state, variables of conduction and parameters, and being able to persist”. Many years later, Holling [6] classified resilience into “engineering resilience” and “ecological resilience” to emphasize the differences between efficiency, stability and predictability on the one hand and persistence, change and unpredictability on the other.
Engineering resilience is characterized by analysing return periods and efficiency by focusing on the recovery capacity of a system in the context of a stable equilibrium. Ecological resilience, in turn, emphasizes the instability that can “move” a system to another regimen of behaviour (also known as the “basin of attraction”).
Based on these concepts, Holling [6] defined resilience as “the magnitude of the disturbance that can be absorbed before the system redefines its functional structure, changing the variables and processes that control behaviour”. Resilience is characterized as the capacity to absorb changes, resist disturbances and maintain functions while focusing on the persistence and robustness of a system in the context of global stability.
Because these different interpretations of resilience were causing confusion, and by arguing that the resilience of a system must be considered in terms of the attributes that control the dynamics of a system. Walker et al. [7] added a third category: socio-ecological resilience, defined as “the potential of a system to tolerate disturbances without collapsing towards a qualitatively different state, maintaining its structure and function, which involves its capacity to reorganize itself, following the changes driven by disturbances”.
Socio-ecological resilience is characterized by analysing the interactions between disturbances, reorganization, sustainability and development in a system and focusing on the capacity to adapt, transform, learn and innovate in a context of unstable equilibrium.
This concept modified existing views that considered systems to be stable by introducing a new perspective that considered the capacity of systems to adapt and change, thus increasing the probability of sustainable development in changing environments where the future is unpredictable.
This broad category integrates resilience with socio-ecologic systems (SES), which integrate social and ecological systems, by focusing not only on the components of both systems but also on their interactions [7].
The SES concept incorporates ideas from fields related to adaptation, robustness and vulnerability by concerning itself with the dynamics and attributes involved in each of these terms, thus becoming broader in scope than any of these individual fields [8,9].
Within the domain of a social system lie subsystems such as culture, politics, the economy, and social organization (society itself); an ecological system domain hosts subsystems such as nature (a setting not created by man) and the environment (a setting created by man) [10].
Folke [11] defined socio-ecological resilience as “an approach or way of thinking that presents a perspective to guide and organize thinking from a broader perspective, providing a valuable framework for the analysis of SES”. This places this field under exploratory research and rapid development, with political implications for sustainable development.
Table 1 synthesizes the major characteristics, perspectives and context of the three concepts of resilience we have identified.
Table 1: Characteristics, perspectives and context of different resilience concepts.
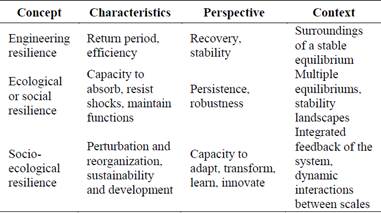
Source: Adapted from [11]
1.2. Resilience for sustainable management of UDS
Different methodologies have been formulated to quantify the concept of resilience; Hosseini et al. [12] classified the procedure for evaluating resilience into qualitative and quantitative methodologies.
The qualitative methodologies include conceptual frameworks (they provide a notion of resilience but do not provide a quantitative value) and semi-quantitative indices (they involve the opinion of experts in their estimation), and the quantitative methodologies include general resilience metrics (they evaluate resilience in the performance of a system) and structural-based models (they evaluate resilience by components).
Based on this classification, Table 2 shows several studies that use the different methodologies to evaluate resilience.
Table 2: Classification of different methodologies used to evaluate resilience.
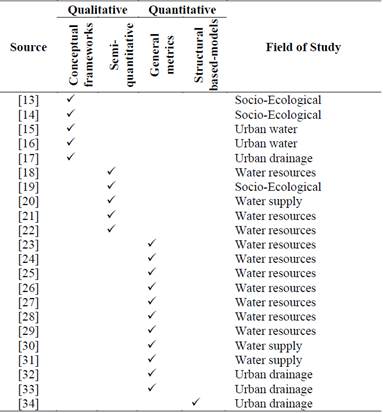
Source: Created by author
Although different methodologies have been proposed to evaluate resilience, there are few studies that have focused on developing the appropriate methodologies to evaluate resilience in UDS [35,36], which makes the study of resilience in this field a novel topic of research for developing quantifiable indicators of resilience that can evaluate all the aspects involved in this concept [16].
To analyse resilience in UDS, conceptual frameworks [17], general resilience metrics [32,33] and structural-based models [34] have been proposed, the majority of which evaluate flood risk in UDS; only one addresses the problem of dragging pollutants [32].
Based on the conceptual perspective of resilience, these frameworks are primarily framed within the concept of engineering resilience. This provides an opportunity to develop new approaches that can evaluate resilience as it applies to UDS and ideally involve an SES from the point of view of socio-ecological resilience; this topic has not been previously addressed, even though there is a wide field of research to be developed.
As a result, this article presents an analysis and related impressions of applying the concept of resilience to the sustainable management of UDS by identifying the various factors, variables and indicators.
2. Methodology
The methodology for the management of scientific information proposed by Gómez et al. [37] was used to identify the variables and indicators of resilience for the sustainable management of UDS.
This methodology consists of four phases: i. Definition of the problem; ii. Search for information; iii. Organization of the information; and iv. Analysis of the information.
To define the problem, we began with the key concepts of sustainability, resilience and SES and their relationship with the sustainable management of UDS. The Scopus database was used to search for information by using keywords such as “sustainability”, “resilience”, “socio-ecological systems” and “urban drainage”, in addition to keywords referring to important problems related to UDS such as “climate change”, “flood risk” and “diffuse pollution”. The period of observation extended from 1979 (when the concept of resilience initially appeared in engineering) until February of 2016.
The following search equations were used: “Resilience AND Socio-Ecological Systems”, “Resilience AND Sustainability OR Urban Drainage”, “Resilience AND Urban Drainage”, “Resilience AND Sustainability AND Climate Change”, “Resilience AND Flood Risk”, “Socio-Ecological Systems AND Sustainability”, “Socio-Ecological Systems AND Climate Change”, “Sustainability AND Urban Drainage”, “Sustainability AND Climate Change OR Urban Drainage”, “Sustainability AND Diffuse Pollution”, “Sustainability AND Flood Risk”, “Urban Drainage AND Climate Change”, “Urban Drainage AND Diffuse Pollution” and “Urban Drainage AND Flood Risk”.
The data mining program RefViz [38] was used to organize the information and select the most relevant articles; the program uses mathematical algorithms to group articles by topic based on the keywords. The results were used to create a concept map denominated as a galaxy in which each topic was grouped based on the frequency of the keywords. The organized information yielded 596 articles of interest forming 24 topic groups.
Once the information was organized, we selected documents and authors for review. After reading the abstracts and conclusions of those articles, an analysis was performed of the articles with the most important ideas and the most relevant aspects for the topic of this study. Once this was completed, were selected four of the 24 groups formed in the information search stage that were of most interest (groups G16, G17, G19 and G21); these included 122 articles. To make this articles selection, it was taken into account that resilience was a main keyword of the document.
The filtered documents were studied in more detail (i.e. it was made a scanning reading of each document) to select those that were relevant for this research (i.e. documents that include all or some factors for the evaluation of resilience in water resources). A total of 19 articles were used to carry out the analysis and comparison of the application of the resilience concept and to identify the common and relevant elements of resilience and the factors, variables and indicators for the sustainable management of UDS.
Fig. 1 shows the groups of interest regarding the topics consulted based on the previously described methodology.
Figure 1: Interest groups in the consulted topics. Period 1979 - February 2016.
3. Results and discussion
3.1. Resilience factors in water resource management
It was determined that resilience was impacted by four factors: i. Flexibility (capacity to change); ii. Resourcefulness (capacity to mobilize resources); iii. Redundancy (presence of options); and iv. Robustness (capacity to resist) [15,39,40].
Table 3 summarizes the description of each of these factors and their adaptation strategies, whereas Table 4 shows how they have been considered in different areas of water resource management.
Table 3: Characteristics of factors considered to evaluate resilience in the management of water resources.
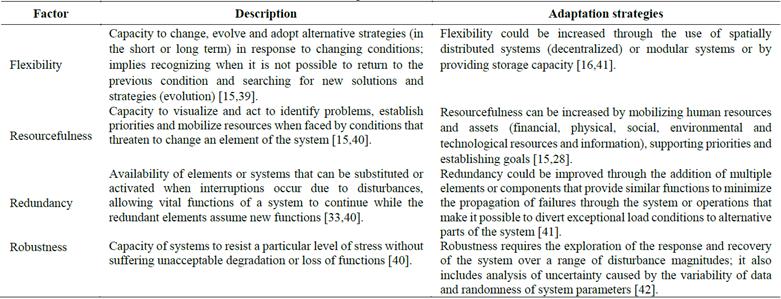
Source: Created by author
Table 4: Factors considered for the evaluation of resilience in different areas of water resource management.
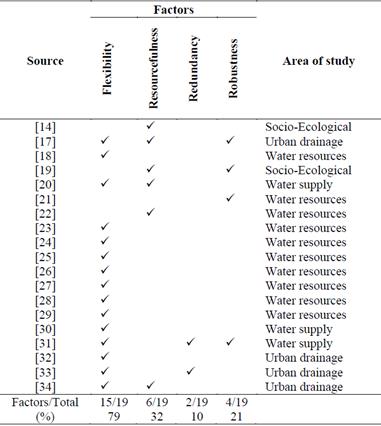
Source: Created by author
Flexibility is the most studied factor because this factor is directly associated with the concept of resilience, which has been changing with the incorporation of additional factors. This factor evaluates the capacity to recover and is associated with the time of failure, reliability and recovery speed of a system from the perspective of risk management. Flexibility has been quantified through semi-quantitative indices that measure the capacity to provide a service [20], mathematical functions that quantify the probability of system failure [23-27,29,32], system reliability [30,31] and indicators based on performance curves of a system that provide information on its behaviour before and after a disturbance [17,18,28,33,34].
Resourcefulness evaluates the availability of economic and social resources and is associated with the capacity to mobilize these resources in adverse conditions. This factor has been quantified through economic variables based on the estimation of damage caused to people and infrastructure [17,18,34], as well as social variables based on economic development, demographic trends, political stability, government policies, market incentives, media organization [14], resource diversity, community and institutional learning, system self-organization [19], governance [20], communication, risk perception, interaction between institutions and risk management policies and tools [22].
Redundancy evaluates the multiplicity of elements that allow the vital functions of a system to continue and is associated with the availability of redundant elements that carry out these functions. This factor has been quantified through metrics such as grouping and meshing coefficients [31], along with an index that combines the magnitude and duration of system failures where redundancy is evaluated by comparing how an existing system functions with and without redundant elements until failure occurs [33]. Of the four identified factors, this is the least considered because of its recent incorporation, which is primarily related to the evaluation of resilience in urban water systems [16,41].
Robustness evaluates the resistance of a system when faced with extreme or unexpected events and is associated with systems that function well, even under uncertain conditions. This factor has been quantified by analysing the resistance capacity of a system by estimating an overload [17] through a variable that addresses change and uncertainty [19]. This is based on a graph that describes the level at which one can establish how a system responds to different levels of disturbance [21] and through metrics such as the central dominance point, the density of articulation points, the density of joints, the spectral void and algebraic connectivity [31].
3.2. Variables and indicators of resilience for sustainable management of UDS
Based on the analysis of these factors, a group of variables and indicators was identified to evaluate the resilience of UDS; these are summarized in Table 5.
Table 5: Variables and indicators used to evaluate the resilience of UDS.
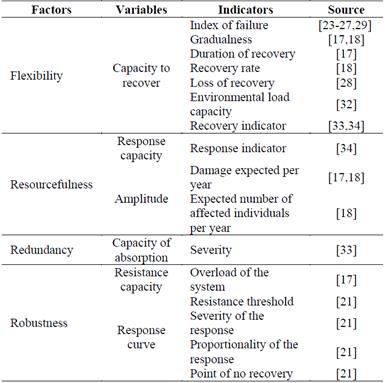
Source: Created by author
Flexibility is associated with the variable of recovery capacity, which evaluates the possibility of a drainage system to return to a normal or stable state after a disturbance; this variable includes several indicators and is the variable most studied by researchers.
The indicators proposed for this variable are the failure index, which quantifies the probability of system failure [23-27,29]; the gradualness, which measures the change in the response of a system with respect to the change of magnitude in a flood surge [17,18]; the recovery duration, which quantifies the time it takes for a system to recover from an unsatisfactory condition [17]; the recovery rate, which measures the recovery rate of the system after a flood [18]; the recovery loss, which quantifies the loss of quality in a system [28]; the environmental load capacity, which quantifies the amount of pollutant emissions that a system can endure [32]; and the recovery indicator, which measures the recovery time from a flood at each node of the system [33,34].
Resourcefulness is associated with two variables. One variable is the response capacity, which evaluates how the components of a drainage system respond to disturbances through a response indicator that allows for estimating the response magnitude in the area surrounding a flooded node [34]. The other variable is the amplitude [35], which evaluates the severity of damage expected in a drainage system after a disturbance through a yearly damage indicator that measures the average damage costs [17,18] and the expected number of affected individuals in a given year [18].
Redundancy is associated with the variable of absorption capacity [43], which evaluates the alternatives that can be offered by a drainage system after the failure of one or more of its component; the key indicator is severity, which measures the magnitude and duration of the maximum failure [33].
Robustness is associated with two variables. One variable is the resistance capacity, which evaluates the magnitude of the damage that a drainage system can endure through a system overload indicator that measures the greatest precipitation intensity that a system can endure [17]. The other variable is the response curve [21], which represents the aspects of robustness applicable to a UDS. The curve shows how a system responds to different disturbance levels through indicators such as the resistance threshold (which measures the point at which the response becomes greater than zero), the severity of the response (which corresponds to the point at which a system is no longer in a normal situation), the proportionality of the response (which relates the response change to the magnitude of the disturbance) and the point of no recovery (which is the point at which a system changes its identity into a new configuration).
Although this study identified resilience factors, variables and indicators in the sustainable management of UDS, continued research exploring other information sources (e.g., other databases besides Scopus, theses, government documents, etc.) is necessary to identify additional elements.
In addition, it will also be necessary to develop more comprehensive conceptual frameworks that can consider all of these factors, variables and indicators and validate them through case studies. However, combination of these factors and what could be the result of this combination is a pending work to be done. The elements identified in this study can serve as a first step in the development of these comprehensive frameworks.
4. Conclusions
The concept of resilience has evolved over the past 40 years, with a diversity of concepts arising from a narrow perspective with specific applications (engineering resilience) to a broader perspective that encompasses a more integral application context (socio-ecological resilience).
The concept of resilience has been scarcely studied as it pertains to UDS management, and the primary application has been in the study of flood risk. Therefore, much remains to be developed in this key field of research.
This study identified four key factors for the evaluation of resilience in water resources: flexibility, resourcefulness, redundancy and robustness. These factors evaluate the capacity to change, the mobilization of resources, the presence of options and the resistance capacity, respectively.
The following variables were identified to analyse resilience in UDS: recovery capacity, response capacity, amplitude, absorption capacity, resistance capacity and response curve. Associated indicators include the index of failure, gradualness, duration of recovery, rate of recovery, loss of recovery, environmental load capacity, recovery indicator, response indicator, expected damage per year, expected number of affected individuals per year, severity, system overload, resistance threshold, response severity, proportionality of response and point of no recovery.
Acknowledgments
The authors wish to express their gratitude for the financial support provided by the Universidad del Valle and Colciencias through Program 617 of 2013 for the financing of National Doctorate Studies granted to the first author and Program 745 of 2016 for the financing of Science, Technology and Innovation Projects and their contribution to the challenges faced by the country.
References
Referencias
Seeliger, L. and Turok, I., Towards sustainable cities: Extending resilience with insights from vulnerability and transition theory. Sustainability, 5(5), pp. 2108-2128, 2013. DOI:10.3390/su5052108
Novotny, E., A new paradigm of sustainable urban drainage and water management, in Oxford Roundtable Workshop on Sustainability, Paper presented at the Oxford Roundtable Workshop on Sustainability - Oxford University, 2008. pp. 1-27.
ONU. Sustainable development goals. 2015. [on line]. [date of reference March 15th 2016]; Available from: http://sustainabledevelopment.un.org/sdgs
Blackmore, J. and Plant, R., Risk and resilience to enhance sustainability with application to urban water systems. Journal of Water Resources Planning and Management [Online]. 134(3), 2008. [date of reference March 2016]. Available at: http://cedb.asce.org/ CEDBsearch/record.jsp?dockey=163932
Holling, C., Resilience and stability of ecological systems. Annual Review of Ecology Evolution and Systematics, 4, pp. 1-23, 1973. DOI: 10.1146/annurev.es.04.110173.000245
Holling, C., Engineering resilience versus ecological resilience [Online], Washington D. C., Peter Schulze – Editor, National Academy of Engineering, 1996 [date of reference March 2016], cap. 4, Engineering within ecological constraints. Available at: http://www.nap.edu/read/4919/chapter/4
Walker, B., Holling, C., Carpenter, S. and Kinzig, A., Resilience, adaptability and transformability in social–ecological systems. Ecology and Society [Online], 9(2), 2004. [date of reference March 2016], Available at: http://www.uu.nl/sites/default/files/resilience-adaptability-and-transformability.pdf
Cumming, G., Spatial resilience in social-ecological system. Cape Town: Springer, 2011.
Lejano, R. and Stokols, D., Social ecology, sustainability, and economics. Ecological Economics, 89, pp. 1-6, 2013. http://dx.doi.org/10.1016/j.ecolecon.2013.01.011
Salas, W, Ríos, L. y Álvarez, J., Bases conceptuales para una clasificación de los sitemas socioecológicos de la investigación en sostenibilidad. Revista Lasallista de Investigación [Online]. 8(2), pp. 136-142, 2011. [date of reference March 2016]. Available at: http://www.scielo.org.co/scielo.php?script=sci_arttext&pid=S1794-44492011000200015
Folke, C. Resilience: The emergence of a perspective for social–ecological systems analyses. Global Environmental Change, 16(3), pp. 253-267, 2006. DOI:10.1016/j.gloenvcha.2006.04.002
Hosseini, S., Barker, K. and Ramirez-Marquez, J., A review of definitions and measures of system resilience. Reliability Engineering & System Safety, 145, pp. 47-61, 2016. DOI: 10.1016/j.ress.2015.08.006
Walker, B., Carpenter, S., Anderies, J., Abel, N., Cumming, G., Janssen, M., Lebel, L., Norberg, J., Peterson, G., Pritchard, R., Resilience management in social-ecological systems: A working hypothesis for a parcipatory approach. Conservation Ecology [Online]. 6(1), 2002. [date of reference March 2016], Available at: http://www.ecologyandsociety.org/vol6/iss1/art14/print.pdf
Ostrom, E., A general framework for analyzing sustainability of social-ecological systems. Science, 325(5939), pp. 419-422, 2009. DOI: 10.1126/science.1172133
da Silva, J., Kernaghan, S. and Luque, A., A systems approach to meeting the challenges of urban climate change. International Journal of Urban Sustainable Development, 4(2), pp. 125-145, 2012. DOI: 10.1080/19463138.2012.718279
Butler, D., Farmani, R., Fu, G., Ward, S., Diao, K. and Astaraie-Imani, M., A new approach to urban water management: Safe and Sure. Procedia Engineering, 89, pp. 347-354, 2014. DOI: 10.1016/j.proeng.2014.11.198
Tahmasebi-Birgani, Y. and Yazdandoost, F., A framework for evaluating the persistence of urban drainage risk management systems. Journal of Hydro-environment Research, 8(4), pp. 330-342, 2014.
de Bruijn, K., Resilience indicators for flood risk management systems of lowland rivers. International Journal of River Basin Management, 2(3), pp. 199-210, 2004. DOI:10.1080/15715124.2004.9635232
Asah, S., Empirical social-ecological system analysis: from theoretical framework to latent variable structural equation model. Environmental Management, 42(6), pp. 1077-1090, 2008. DOI:10.1007/s00267-008-9172-9
Milman, A. and Short, A., Incorporating resilience into sustainability indicators: An example for the urban water sector. Global Environmental Change, 18, pp. 758-767, 2008. DOI:10.1016/j.gloenvcha.2008.08.002
Mens, M., Klijn, F., Bruijn, K. and van Beek, E., The meaning of system robustness for flood risk management. Environmental Science & Policy, 14(8), pp. 1121-1131, 2011. DOI: 10.1016/j.envsci.2011.08.003
Schelfaut, K., Pannemans, B., van der Craats, I., Krywkow, J., Mysiak, J. and Cools, J., Bringing flood resilience into practice: The FREEMAN project. Environmental Science and Policy, 14(7), pp. 825-833, 2011. DOI: 10.1016/j.envsci.2011.02.009
Hashimoto, T., Stedinger, J. and Loucks, D., Reliability, resiliency, and vulnerability criteria for water resource system performance evaluation. Water Resources Research, 18(1), pp. 14-20, 1982. DOI: 10.1029/WR018i001p00014
Moy, W., Cohon, J. and ReVelle, C., A programming model for analysis of the reliability, resilience, and vulnerability of a water supply reservoir. Water Resources Research, 22(4): pp. 489-498, 1986. DOI: 10.1029/WR022i004p00489
Maier, H., Lence, B., Tolson, B. and Foschi, R., First-order reliability method for estimating reliability, vulnerability, and resilience. Water Resources Research, 37(3), pp. 779-790, 2001. DOI: 10.1029/2000WR900329
Kjeldsen, T. and Rosbjerg, D., Choice of reliability, resilience and vulnerability estimators for risk assessments of water resources systems. Hydrological Sciences Journal, 49(5), pp. 755-767, 2004. DOI: 10.1623/hysj.49.5.755.55136
Li, Y. and Lence, B., Estimating resilience for water resources systems. Water Resources Research, 43(7), 2007. DOI: 10.1029/2006WR005636
Wang, C. and Blackmore, J., Resilience concepts for water resource systems. Journal of Water Resources Planning and Management,
(6), pp. 528-536, 2009. DOI: 10.1061/(ASCE)0733-9496(2009)135:6(528)
Liu, D., Chen, X. and Nakato, T., Resilience assessment of water resources system. Water Resources Management, 26(13), pp. 3743-3755, 2012. DOI: 10.1007/s11269-012-0100-7
Todini, E., Looped water distribution networks design using a resilience index based heuristic approach. Urban Water, 2(2), pp. 115-122, 2000. DOI: 10.1016/S1462-0758(00)00049-2
Yazdani, A., Otoo, R. and Jeffrey, P., Resilience enhancing expansion strategies for water distribution systems: A network theory approach. Environmental Modelling & Software, 26(12), pp. 1574-1582, 2011. DOI: 10.1016/j.envsoft.2011.07.016
Ning, X., Liu, Y., Chen, J., Dong, X., li, W. and Liang, B., Sustainability of urban drainage management: a perspective on infrastructure resilience and thresholds. Frontiers of Environmental Science & Engineering, 7(5), pp. 658-668, 2013. DOI: 10.1007/s11783-013-0546-8
Mugume, S., Gomez, D., Fu, G., Farmani, R. and Butler, D., A global analysis approach for investigating structural resilience in urban drainage systems. Water Research, 81, pp. 15-26, 2015. DOI: 10.1016/j.watres.2015.05.030
Birgani, Y. and Yazdandoost, F., Resilience in urban drainage risk management systems, Proceedings of the ICE - Water Management, 2015. pp. 1-13.
de Bruijn, K., Resilience and flood risk management. Water Policy [Online]. 6(1), 2004. [date of reference March 2016]. Available at: http://wp.iwaponline.com/content/6/1/53
Mugume, S., Goméz, D. and Butler, D., Quantifying the resilience of urban drainage systems using a hydraulic performance assessment approach, 13th International Conference on Urban Drainage, 2014. pp. 1-9.
Gómez-Luna, E., Navas, D., Aponte-Mayor, G. y Betancourt-Buitrago, L., Metodología para la revisión bibliográfica y la gestión de información de temas científicos, a través de su estructuración y sistematización. DYNA [Online]. 81(184), 2014. [date of reference March 2016]. Available at: http://www.redalyc.org/ articulo.oa?id=49630405022
Agrawal, A., Using RefViz© with EndNote [Online]. Springer Science + Business Media, 2006 [date of reference March 2016], cap. 12, Endnote 1-2-3 Easy!: Reference Management for the Professional. Available at: http://link.springer.com/chapter/10.1007%2F978-0-387-95901-6_12
Bruneau, M. and Reinhorn, A., Exploring the concept of seismic resilience for acute care facilities. Earthquake Spectra, 23(1), pp. 41-62, 2007. DOI: 10.1193/1.2431396
Pandit, A., Resilience of urban water systems: An 'Infrastructure Ecology' approach to Sustainable and Resilient (SuRe) planning and design, Ph.D. Thesis, School of Civil and Environmental Engineering, Georgia Institute of Technology, Atlanta, USA, 2014.
Mugume, S., Diao, K., Astaraie-Imani, M., Fu, G., Farmani, R. and Butler, D., Enhancing resilience in urban water systems for future cities. Water Science and Technology: Water Supply, 15(6), pp. 1343-1352, 2015. DOI: 10.2166/ws.2015.098
Anderies, J., Folke, C., Walker, B. and Ostrom, E., Aligning key concepts for global change policy: Robustness, resilience, and sustainability. Ecology and Society, 18(2), 8, 2013. DOI: 10.5751/ES-05178-180208
Balsells, M., Barroca, B., Amdal, J., Diab, Y., Becue V. and Serre, D., Analysing urban resilience through alternative stormwater management options: Application of the conceptual Spatial Decision Support System model at the neighbourhood scale. Water Science & Technology, 68(11), pp. 2448-2457, 2013. DOI: 10.2166/wst.2013.527
Cómo citar
IEEE
ACM
ACS
APA
ABNT
Chicago
Harvard
MLA
Turabian
Vancouver
Descargar cita
CrossRef Cited-by
1. Ploutarchos N. Kerpelis, Dimitrios E. Alexakis, Spyridon K. Golfinopoulos. (2022). A Qualitative Approach to the Seismic Estimation of Wastewater Treatment Plants and Potential Impacts on the Hydrosphere. Water, 14(20), p.3225. https://doi.org/10.3390/w14203225.
2. Marta Marçal Gonçalves, Francisco Villena-Manzanares. (2023). The role of human factor for urban infrastructures. PROCEEDINGS OF THE 15TH INTERNATIONAL CONFERENCE ON X-RAY MICROSCOPY – XRM2022. PROCEEDINGS OF THE 15TH INTERNATIONAL CONFERENCE ON X-RAY MICROSCOPY – XRM2022. 2990, p.130007. https://doi.org/10.1063/5.0170386.
3. Jiahui Lu, Jiahong Liu, Xin Su. (2025). A resilience evaluation method considering functional and structural resilience of urban drainage systems. Urban Water Journal, 22(4), p.372. https://doi.org/10.1080/1573062X.2025.2472327.
4. João Barreiro, Filipa Ferreira, Rita Salgado Brito, José Saldanha Matos. (2024). Development of Resilience Framework and Respective Tool for Urban Stormwater Services. Sustainability, 16(3), p.1316. https://doi.org/10.3390/su16031316.
Dimensions
PlumX
Visitas a la página del resumen del artículo
Descargas
Licencia
Derechos de autor 2017 DYNA

Esta obra está bajo una licencia internacional Creative Commons Atribución-NoComercial-SinDerivadas 4.0.
El autor o autores de un artículo aceptado para publicación en cualquiera de las revistas editadas por la facultad de Minas cederán la totalidad de los derechos patrimoniales a la Universidad Nacional de Colombia de manera gratuita, dentro de los cuáles se incluyen: el derecho a editar, publicar, reproducir y distribuir tanto en medios impresos como digitales, además de incluir en artículo en índices internacionales y/o bases de datos, de igual manera, se faculta a la editorial para utilizar las imágenes, tablas y/o cualquier material gráfico presentado en el artículo para el diseño de carátulas o posters de la misma revista.